Melting of Ice Sheets
Over the past decade, the Antarctic and Greenland Ice Sheets have been loosing its mass at an alarming rate. Antarctic ice-shelves are melting by turbulent transport of heat and salt to the ice face, predominantly under the influence of warmer Circumpolar Deep Water entering ice shelf cavities from the surrounding...
Project status
Content navigation
About
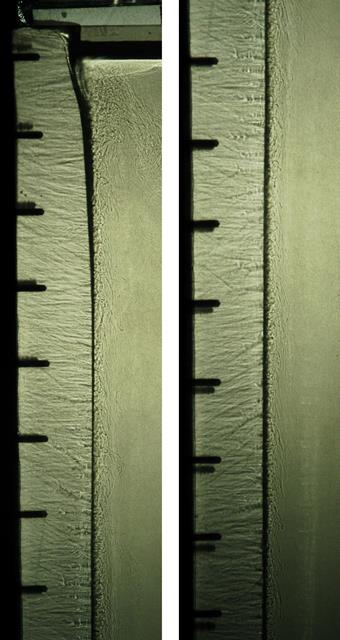
Over the past decade, the Antarctic and Greenland Ice Sheets have been losing its mass at an alarming rate. Antarctic ice-shelves are melting by turbulent transport of heat and salt to the ice face, predominantly under the influence of warmer Circumpolar Deep Water entering ice shelf cavities from the surrounding Southern Ocean. This mass loss occurs largely from the underside and fronts of ice shelves where glaciers reach the ocean and from the icebergs that calve from the shelves. The water exiting ice shelf cavities contributes to Antarctic Bottom Water, a crucial component of the global thermohaline circulation. Knowledge of these processes, and the feedbacks between them, is needed to predict future climate change.
We are investigating the physical processes that control these ice-ocean interactions and the resulting circulation at basin scale. Group research focuses on:
Laboratory models
The laboratory experiments are being conducted in our temperature controlled cold room. The cold room allows us to conduct experiments at conditions that are close to what would be found near actual Antarctic ice shelves. The laboratory experiments let us measure some of the fluid properties a lot closer to the ice surface than a lot of the field studies can as well as in a more controlled environment. They also provide a real data set that can be used to confirm and test theoretical or numerical results. We have currently been focused on the effect of ambient temperature and stratification on the melt rate but expect to add external flow and geometry in the future
Numerical models
Melting (or dissolving) of the ice involves complex turbulent boundary layer flow, which is largely driven by convection due to temperature and salinity differences. Direct Numerical Simulation (DNS) and Large Eddy Simulation (LES) are excellent tools for obtaining solutions under both laboratory and ocean conditions that can be compared with experimental data and, eventually, ocean observations. Present numerical results for a small-scale ice interfaces successfully show the formation of a meltwater boundary layer and multiple quasi-horizontal thermohaline layers spreading across the cavity, consistent with laboratory experiments. An example is shown below; the warmer water in blue is the turbulent meltwater flowing up the inclined ice interface.
Recent developments in regional and global ocean modelling have enabled the inclusion of the ocean cavities beneath ice shelves in models. However, a lack of direct observations in these remote, ice-covered regions have limited our understanding of melt, hampered by complex physical processes that occur at the ice-ocean boundary layer on small temporal and spatial scales. The development of parameterisations of ice shelf melt has been underway for some time but melt rates across Antarctica still remain uncertain. We aim to incorporate findings of physical processes from high-resolution numerical models and laboratory studies to refine melt parameterisations in global ocean models.